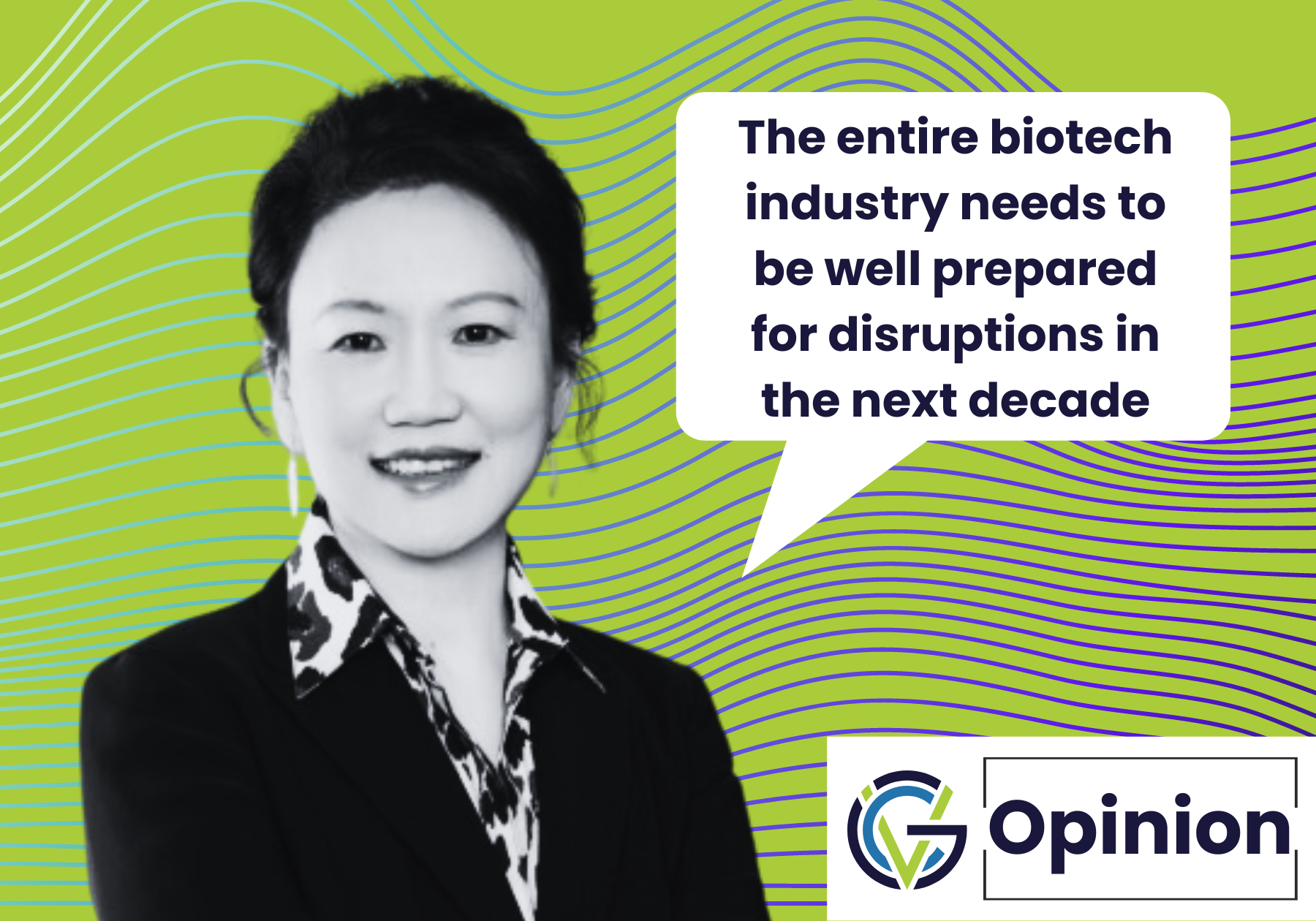
In March 2023, the US government announced an ambitious new course for the US national biostrategy.
The US bioeconomy — which includes everything from creating vaccines to using biological systems to produce materials such as plastic and fuels — is booming. An article in Forbes in September 2022 notes that this sector is valued at nearly a trillion dollars and predicted to grow globally to $30trn over the next two decades.
The US Executive Order to advance biotechnology and biomanufacturing seeks to strengthen supply chains, address health issues and tackle climate change. It outlined several key targets including:
1) Climate: in 20 years to displace 90% of today’s plastics and other commercial polymers with polymers made from bio-based feedstocks.
2) Food and agriculture: By 2030, to reduce greenhouse gas emissions by 50% in the US and 30% globally.
3) Supply chain: To supply more than 30% of the US chemical demands via sustainable and cost-effective bio-manufacturing within 20 years,
4) Health: In 20 years to reduce the manufacturing cost of cell-based therapies 10-fold.
5) Cross-cutting advantages: in 5 years, sequence the genomes of 1 million microbial species and understand the functions of more than 80% newly discovered genes.
East-west biotech battle
But the US is not the only country putting resources into the bio-economy. Michael A Fisher, Senior Fellow at the Federation of American Scientists, a nonprofit global policy think tank warns that the US could lose ground to countries like China.
“China aims to dominate the 21st-century bioeconomy and has prioritized growth of its bioeconomy in its five-year plans. From 2016 to July 2021, the market value of publicly listed biopharmaceutical innovators from China increased approximately 127-fold across several major stock exchanges, to more than $380 billion, with biotechnology companies accounting for more than 47 percent of that valuation,” he says in the same Forbes article.
“The biotech and bio-manufacturing space is the next big race between the east and the west.”
The biotech and bio-manufacturing space is the next big race between the east and the west. In terms of the materials arena, the entire industry needs to be well prepared for disruptions in the next decade or two.
Challenges in meeting biotech goals
Meeting the goals set out in the bio-economy strategy will be challenging. For example, of we want to replace 90% of today’s plastics with bio-manufacturing, chemical sector leaders such as BASF, SABIC, Arkema and others will have to substantially change their industrial processes. For at least 30% of the chemical demand in the supply chain to come from bio-manufacturing, plans for bio-based resources need to be put in place from today.
To sequence 1 million microbial species in 5 years and to have 80% of these functions understood means the entire bio-engineering process needs to be accelerated with technology.
Industry is facing an entire paradigm shift
Take plastics manufacturing. Currently, oil as a feedstock for plastics is cheaper than using bio-engineered polymers. For many consumer brands, oil-based plastics packaging will always be their first choice. Change takes time, and hopefully 20 years will be enough to drive down prices: this calls for breakthroughs in research to develop a cost-effective, mass-produced feedstock.
In western markets, there is a wide selection of synbio startups driving to alter conventional products and processes, transforming the material world, and contributing to the bio-manufacturing industry, from food, to cosmetics, to health and wellness – but none are profitable at this stage.
Synthetic biology accelerates with tech
Synthetic biology, or synbio, is driving many of these global biotech and bio manufacturing goals.
Synthetic biology processes are based on engineerin plant, animal or microbe cells to effectively turn into “cell factories”. The more researchers learn about genomics, the more they can program DNA; much like software engineers can program computers, according to a report by Barclays.
Synbio uses genetic engineering to alter a cell’s DNA to make target molecules for manufacturing use across industries. It plays a role in healthcare, has shown promise in energy and is gaining traction in the consumer goods space.
Synbio has been attracting an increasing amount of venture capital, especially in the last few years. One of the reasons why it’s gaining traction is due to the progress of computing power. Bioinformatics, with computer science, the power of AI and machine learning, combined with chemistry, physics, molecular biology, agriculture and engineering science, work together to create new bio-based solutions.
Synbio companies are in a Design, Build Test and Learn (DBTL) development cycle. Unlike conventional chemistry, this area with living organisms. In the R&D phase, scientists spend time and effort to develop, test and study the effect of altering genomes, microbes, enzymes, to eventually synthetically engineer them.
New genome-editing technologies, such as CRISPR-Cas9, are helping the creation of novel DNA combinations, reducing the costs of editing DNA, and increasing the length of DNA strands that can be replicated without error. It has also become possible to carry out synbio without the use of live cells, using just metabolic cell processes, speeding up the testing process.
Through synbio, supply might no longer be constrained by the availability of raw materials. As a BCG publication says: “Companies can engineer and manufacture an infinite quantity of things, cell by cell, from scratch. Half a gram of cattle muscle could create as much as 4.4 billion pounds of beef—more than Mexico consumes in a year.”
While synbio might seem to be a magic wand to pursue a fully organic, sustainable and low-carbon future, challenges remain in its ‘need for speed’.
Two methods of development in Synbio: full-cell or cell-free
There are two ways to pursue synbio development to engineer bio-materials – through live cells or synthetic cells, also known as: full-cell or cell-free.
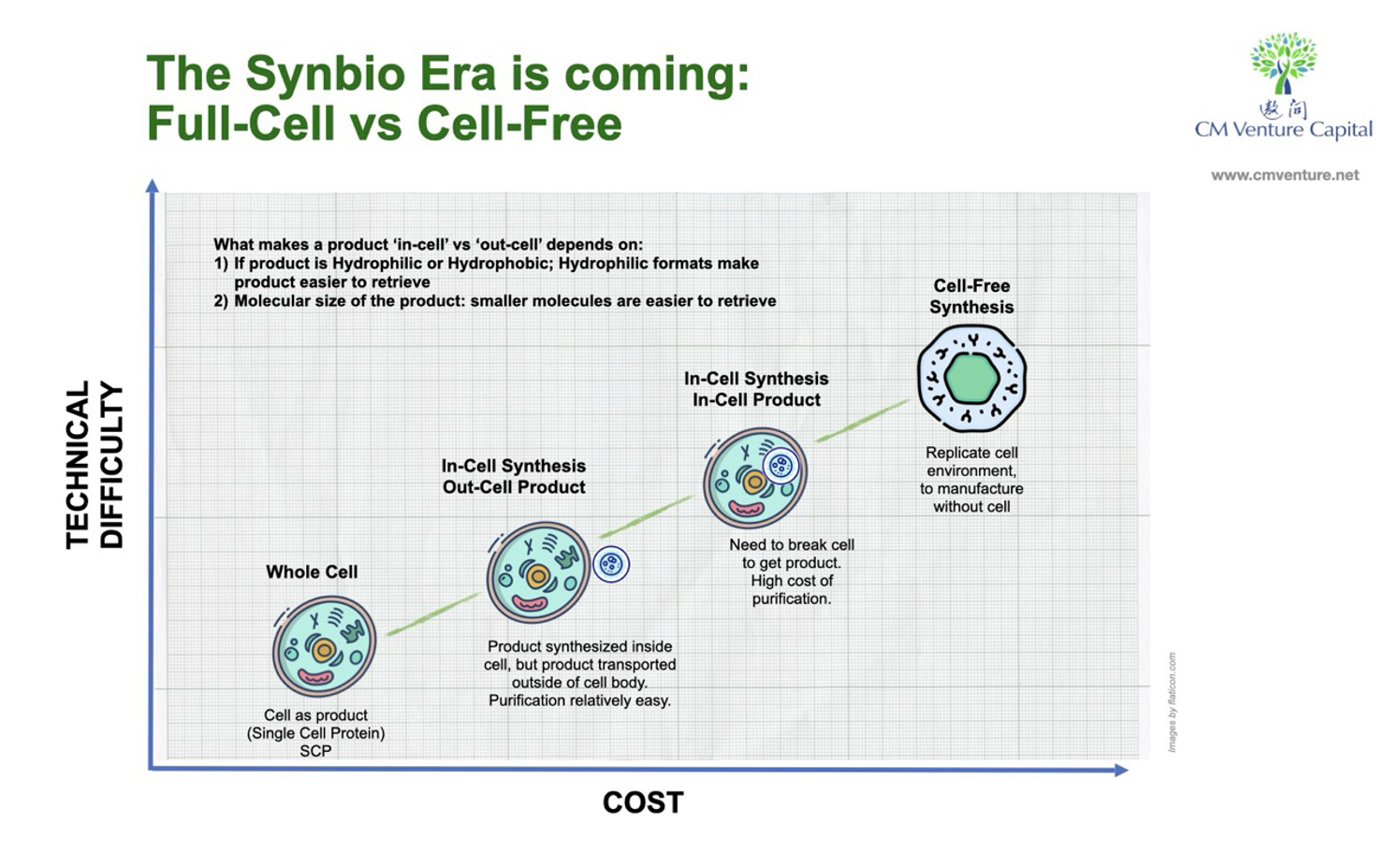
(1) Full cell (in vivo/live cells)
Using live cells (full-cell) in the traditional fermentation process, scientists start with the design, then building the microbes, testing them and then inspecting/recording the results. The whole cycle repeats itself until desired results are achieved – or if not, another design will be explored.
In the conventional full-cell process, there is a limit to the volume of microbes you can place in reactors. The fermentation process is complex, influenced by the control of culture and process parameters. If the control isn’t managed well, batches may fail, affecting amplification.
This means there’s a strict limitation on the amount of chemicals that can be produced on each course. After fermentation, the cells need to be broken down, and then purified to extract results. It’s very complex, labor and time intensive, so costs are very high.
The full-cell method needs to keep cells alive for the entire development process. There’s also a need to isolate the product from rest of the cell (as cells have their own processes to keep itself alive) through a process called protein purification, and it’s limited to products that aren’t toxic to the cells.
The other way is to make the process as inorganic as possible, also known as the ‘cell-free’ path.
(2) Cell-free (in vitro/synthetic cells)
The new frontier in accelerating synbio development is the cell-free process. You can conduct the same process without the microbial environment – with less time and less complexity. But a few issues remain, one of which is its high cost.
Cell-free systems can generally be defined as platforms where biochemical reactions occur independently of living cells. Cell-free systems are divided into two types based on preparation method: extract-based and enzyme-based.
The cell-free process is a synthetic process with replicates the basic functions of a living cell – in scientist terms, there’s no limit to the monomers — sugars, amino acids, fatty acids and nucleotides — you can convert into more complex polymers. Instead of, say a traditional 20% output with full-cell (organic process), the cell-free process allows researchers to achieve as much as 90% output (inorganic process). Cell-free platforms are not restricted by limits for supporting life.
For example, scientists generally need to study membrane proteins to develop models. Membranes and proteins are co-existential, meaning both need to be present in research, as it’s based on the organic format of living bodies. If both elements are separate, the model won’t develop in the way they should in life. However, the cell-free process allows researchers to study/develop the proteins without membranes.
So, with cell-free formats, scientists can easily control and access protein synthesis with in-vitro bio systems without membranes, and achieve metabolic manipulation to enable inexpensive ATP generation, or incorporate unnatural amino acids (useful for NMR studies). The cell-free synthesis is protein synthesis using recombinant elements – a ‘method of synthesis’ is to make proteins for use in research.
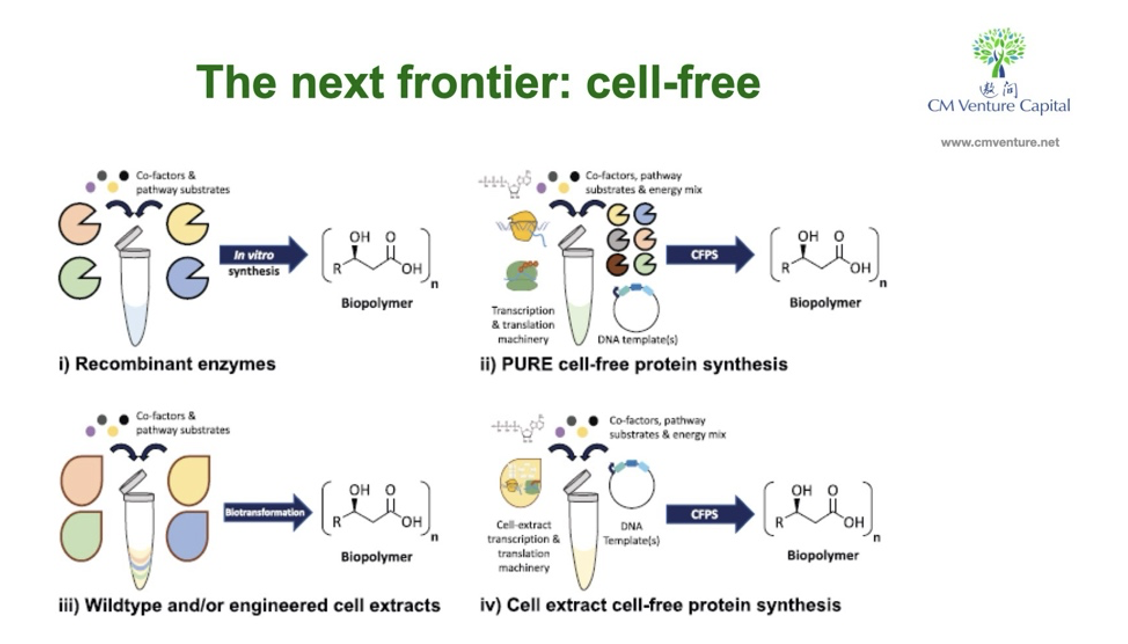
Comparing the two formats
With the cell-free platform, the Design, Build, Test, Learning (DBTL process) remains the same, but is unencumbered by the organic requirements of full-cell formats. With reference from the National Library of Medicine, this chart clearly shows the differences between a cell-free and full-cell process:
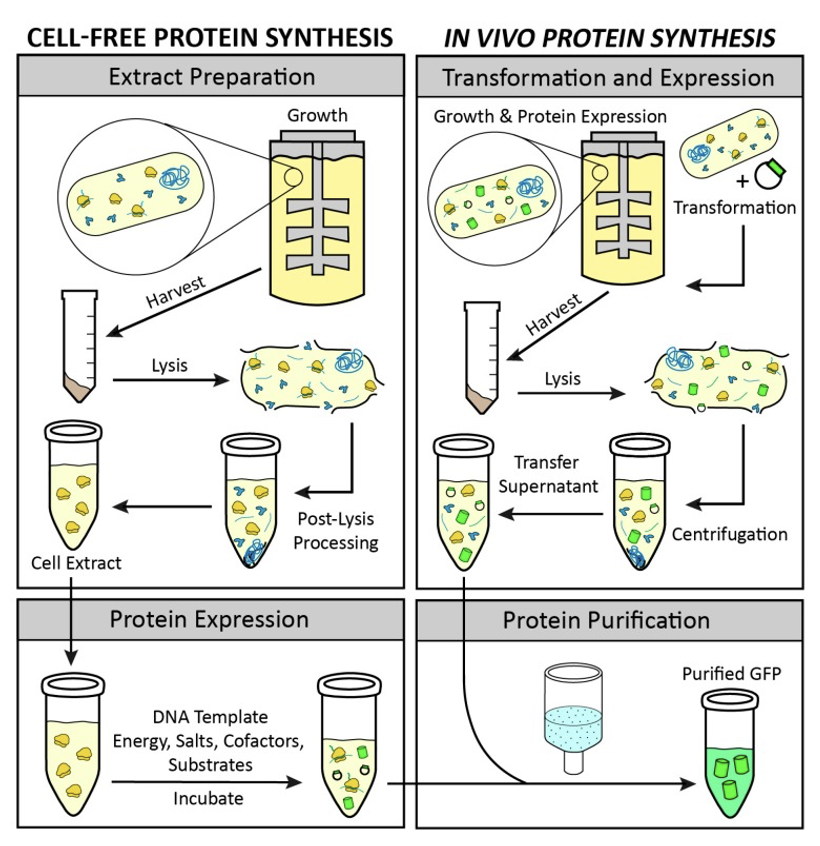
“A comparison of cell-free and in vivo protein synthesis methods. Through visualization of the main steps of in vitro and in vivo protein expression, the advantages of cell-free protein synthesis emerge. These include the elimination of the transformation step, an open reaction for direct manipulation of the environment of protein production, the lack of constraints based on the cell’s life objectives, the channeling of all energy toward production of the protein of interest, and the ability to store extracts for on-demand protein expression. Green cylinders represent synthesized green fluorescent protein (GFP).”
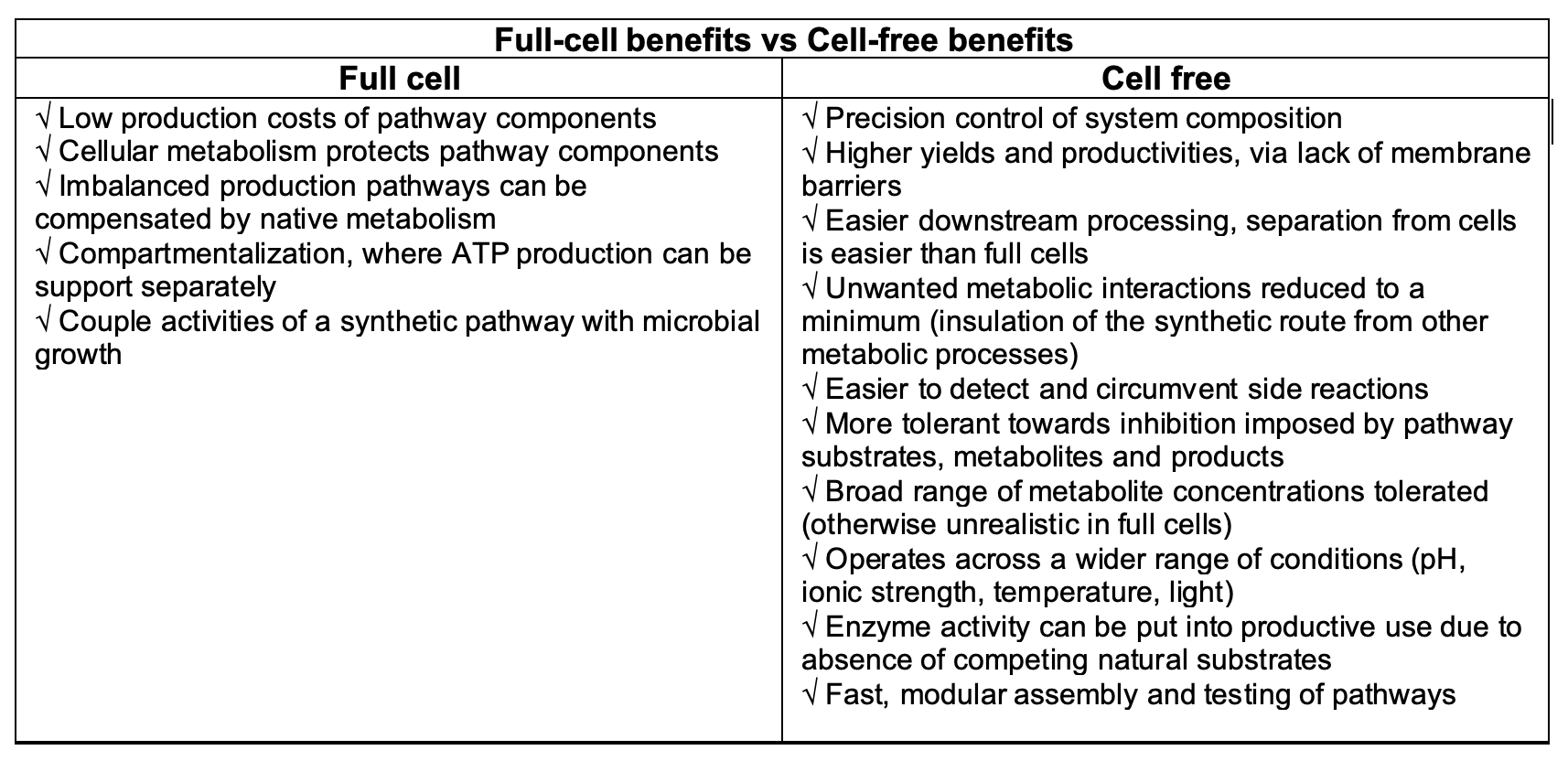
It’s clear there are more benefits to the cell-free format. Yet the full-cell format its own unique strengths, the most outstanding being its low cost. Of course, one way is to accept and combine both processes: synthetic pathways can be designed, tested, and optimised in cell-free systems first, then transplanted to living cells for further optimisation next, and then eventually upscaled.
For products in the synbio industry to succeed, two areas – as mentioned in a BCG report – must be considered:
1. Time to market
(1a) Scale. Once the technology is proven in a lab, then at scale in a bioreactor, it’s a milestone. Over 90% of synbio breakthroughs fail because they can’t be scaled. Different cell types react differently to scaling. Take for example: yeast in bioreactors requires a scale of at least 600 kiloliters a year, while animals cells attains scale at just 45 kiloliters. Scaling takes time and effort. The industry practice is to execute 1,000 run-time hours for any change to a process.
(1b) Cost. The startup’s aim is to lower the cost of manufacturing or process to less than the reference/target material (ie. bioplastic vs oil-based plastic). A cost-saving method is to keep the facility close to feedstock sources, for example.
2. Diffusion
This is about how well-accepted the new technology is. It’s a combination of national policies, industry concentration, investments, nature of product, its scarcity, and so on. Diffusion also depends on the the supporting ecosystem’s maturity: such as scientific talents, academic partners, and supply chains.
The Chinese synbio space
Aside from studying the global biotech companies, we’ve also looked at dozens of startups in the Chinese synbio industry. From the data, we zoned the Chinese synbio startups into two areas: platform vs products, and those which have been in the market for around 5 years.
We’ve seen that there are no companies doing just pure platforms. All the Chinese synbio startups are running platforms and products, even if their platforms remain as their core expertise. The reason behind this is a simple matter of survival, since research and breakthroughs take time. Typically, it takes over 5 years to properly research and develop what can be done with a new discovery.
And while there are Chinese synbio companies spanning the cell-free and full-cell formats, we haven’t encountered any startup in the cell-free space with a more than $500m product.
There is great potential in the cell-free format to deliver a wider array of possibilities for the materials industry. But the greatest remaining hurdle is cost. Full cell formats remain much more affordable and available for research and development, even if they’re cumbersome and time-consuming to handle – part of the reason behind the delays to advancements in bio-material developmental.
Synbio is in its own ‘DBTL phase’
So, it seems the entire Synbio industry itself is in the Design, Build, Test, Learn phase – cycling until it’s able to achieve breakneck efficiencies to deliver the breakthroughs needed to support the bio economy in the twenty years or less. But with constant advances in AI, computing technology, production techniques, and most importantly, embracing the cell-free format, achieving the bold goals doesn’t have to be a far-fetched dream.
Excerpts by Dr Daniel Lukamto, formerly A*Star Singapore
Dr. Min Zhou is the CEO of CM Venture Capital, a China-headquartered investment company which partners with a number of multinationals to help them invest in cutting-edge hardtech.